I received a question via reddit a few moments ago, and I think the answer might be of general interest, so I decided to answer it here:
“Would you happen to know the effective or perceived resolution of the [Valve Index headset] when viewing a 50″ virtual screen from say.. 5 feet away? Do you think its equivalent to a 50″ 1080p tv from 5 ft away yet? I was also wondering why when I look at close up objects on the index that I can see basically no screen door effect, but when looking into the distance at the sky then suddenly the sde becomes very noticeable.”
Okay, so that’s actually two questions. Let’s start with the first one, and do the math.
The first thing we have to figure out is the resolution of a 50″ 1080p TV from 5 feet away. That’s pretty straightforward: a 1080p TV has 1920 pixels horizontally and 1080 pixels vertically. Meaning, it has √(19202 + 10802) = 2202.9 pixels along the diagonal, and – assuming the pixels are square – a pixel size of 50″/2202.9 = 0.0227″. Next we have to figure out the angle subtended by one of those pixels, when seen from 5 feet away. That’s α = tan-1(0.0227″/(5⋅12″)) = 0.0217°. Inverting that number yields the TV’s resolution as 46.14 pixels/°.
Figuring out a VR headset’s resolution is more complex, and I still haven’t measured a Valve Index, but I estimate its resolution in the forward direction somewhere around 15 pixels/°. That means the resolution of the hypothetical 50″ TV, viewed from 5 feet away, is approximately three times as high as the resolution of a Valve Index. The interested reader can simulate the perceived resolution of a VR headset of known resolution by following the steps in this article.
The second question is about screen-door effect (SDE). As shown in Figure 1, SDE is a high-frequency grid superimposed over a low-frequency (low-resolution) pixel grid, which makes it so noticeable and annoying. But why does it become less noticeable or even disappear when viewing virtual objects that are close to the viewer? That’s vergence-accommodation conflict rearing its typically ugly, but in this case beneficial, head. When viewing a close-by virtual object, the viewer’s eyes accommodate to focus on a close distance, but the virtual image shown by the VR headset is still at its fixed distance, somewhere around 1.5‒2m away depending on headset model. Meaning, the image will be somewhat blurred, and SDE, being a high-frequency signal, will be affected much more than the lower-frequency actual image signal.
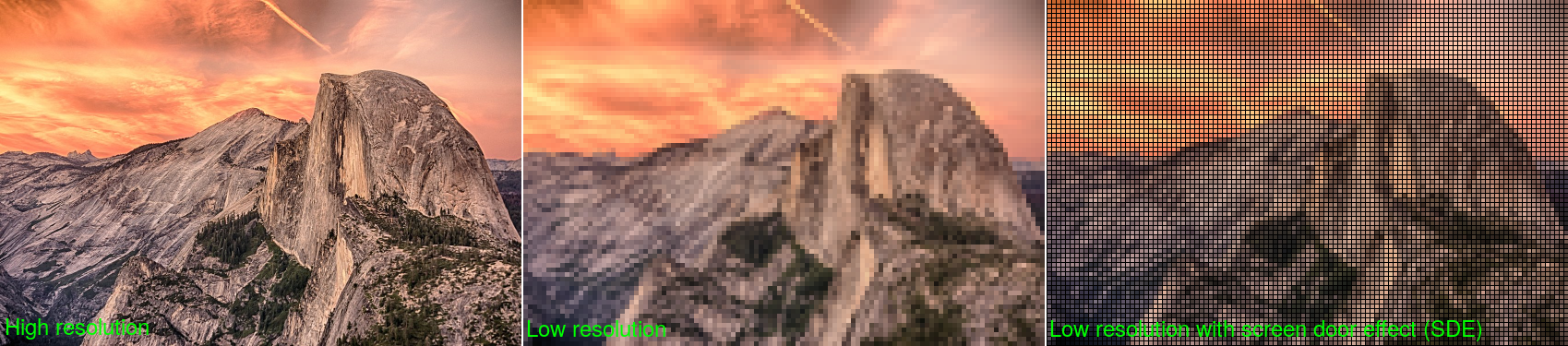